What drives the relentless pursuit of knowledge, especially in the face of seemingly insurmountable challenges? Understanding this fundamental drive is crucial for appreciating the intellectual and scientific achievements of significant figures.
This pursuit, this relentless drive to unravel the universe's mysteries, is often represented by individuals who dedicated their lives to profound research. Their work, often groundbreaking, often challenges established paradigms and pushes the boundaries of human understanding. Examples range from astronomical observations to complex theoretical frameworks. The relentless pursuit of knowledge is not merely an intellectual exercise; it shapes our understanding of the world and our place within it.
Such dedication, often spanning decades, fosters innovations in various fields. The accumulated knowledge, insights, and discoveries generated by these individuals form the bedrock of modern scientific and technological progress. The impact of their relentless investigations often echoes through generations, profoundly influencing subsequent generations of researchers. The pursuit of knowledge is often intertwined with a desire to understand, explain, and ultimately to improve the human condition. This relentless inquiry is what fuels the progress of civilization.
- Elevate Your Style With Ralph Lauren Outlet Online Big And Tall
- Understanding The Walk Your Plans Cost An Indepth Guide
Moving forward, this article will delve into the significance of such dedication. It will examine the specific contributions of various individuals to the advancement of scientific and intellectual discourse, including the impact of their theoretical frameworks and empirical investigations.
Hawking
Understanding the multifaceted nature of this pursuit requires exploring its various dimensions. This exploration encompasses theoretical frameworks, observational methodologies, and societal impact. Key aspects of this intellectual endeavor are crucial to appreciating its significance.
- Theoretical models
- Observational evidence
- Mathematical rigor
- Scientific methodology
- Predictive accuracy
- Societal influence
- Accessibility to knowledge
These aspects highlight the complex interplay between abstract reasoning, empirical data, and practical application. Theoretical models, like those developed by renowned physicists, attempt to explain intricate phenomena, requiring rigorous mathematical frameworks. Observational evidence, such as astronomical data, validates or refutes these models. A high degree of predictive accuracy further underscores the robustness of the scientific method. The societal influence of such research is profound, shaping not only scientific advancements but public understanding of fundamental concepts. Accessibility to knowledge, facilitated by publications and educational initiatives, fosters a continuous cycle of discovery and progress, exemplified by the widespread dissemination of Stephen Hawking's work.
- Innovative Kitchen Design The Pot Filler Under Microwave
- Discovering The Refreshing World Of Crystal Light Fruit Punch Ingredients
1. Theoretical Models
Theoretical models play a pivotal role in scientific inquiry, particularly in fields like cosmology and physics. These models, often abstract representations of complex phenomena, offer frameworks for understanding the universe and its constituents. The development and refinement of theoretical models, in the pursuit of deeper understanding, are intrinsically linked to significant figures in science. Their importance lies in providing a means to make predictions, suggest novel experiments, and ultimately, enhance our comprehension of reality.
- Mathematical Formalisms
Mathematical frameworks are fundamental to theoretical modeling. Equations and systems of equations are used to describe physical laws and relationships between variables. For instance, Einstein's theory of general relativity employs complex mathematical models to explain gravity as a curvature of spacetime. This approach underpins many theoretical models in the scientific domain, and is crucial in the pursuit of understanding.
- Predictive Capabilities
A critical aspect of a robust theoretical model is its ability to make testable predictions about observable phenomena. The ability to predict previously unknown observations provides compelling evidence for the validity of a model. For example, predictions based on models of black holes, made theoretically, later found observational confirmation. This strengthens the model and its ability to offer a more accurate understanding of the subject matter.
- Explanatory Power
Theoretical models offer explanations for observed patterns and events. These explanations, based on underlying principles and relationships, provide a means of interpreting data and constructing a comprehensive understanding of a given phenomenon. Models relating to cosmic evolution, for example, strive to explain the origin and development of the universe.
- Evolution and Refinement
Theoretical models are rarely static. As new data emerge or existing models encounter limitations, they undergo refinement and adaptation. This dynamic process, central to scientific progress, underscores the iterative nature of scientific inquiry. Models evolve over time, in response to the accumulation of new evidence and challenges to existing understanding. The evolution of models is a hallmark of the scientific method.
The development and application of theoretical models are inherent to the scientific pursuit. Through rigorous mathematical structures and the pursuit of explanatory power, models furnish frameworks for understanding. Their predictive capacity, coupled with their adaptive evolution, underpins scientific progress. In the quest for comprehension, models provide crucial tools for grasping fundamental concepts, driving discovery, and furthering our understanding of the universe.
2. Observational Evidence
Observational evidence plays a critical role in validating or refining theoretical models, including those proposed in the field of theoretical physics. The acquisition and interpretation of observational data are essential components of the scientific method. This process often involves meticulously collecting data from various sources, meticulously analyzing patterns and anomalies, and drawing conclusions supported by empirical evidence. The significance of observational evidence stems from its ability to constrain and refine theoretical frameworks. Without robust observational data, theoretical models risk becoming detached from reality.
Consider the investigation of black holes. Theoretical models predicted the existence of such objects, yet direct observation was necessary to confirm these predictions. Event horizons, the region around a black hole from which nothing can escape, were postulated theoretically. However, observational evidence in the form of gravitational wave detections, produced by merging black holes, and observations of stellar orbits around supermassive black holes, provided crucial confirmation of these theoretical concepts. This convergence of theory and observation enhances the reliability and comprehensiveness of scientific understanding. Further, advancements in observational technology allow for more detailed observation, leading to refined theoretical models and an increasingly accurate understanding of black hole formation and characteristics. These observations help refine existing theories and lead to the development of new ones, contributing to the continuous evolution of the field.
In essence, observational evidence provides the empirical grounding necessary for validating theoretical frameworks. Without this connection to the real world, theories remain speculative. The detailed examination of observational data, combined with theoretical modeling, provides a comprehensive understanding of phenomena, leading to a more nuanced and reliable understanding of the natural world. Future advancements in observational technology and methodologies will undoubtedly continue to reveal new insights, further enriching our knowledge and challenging our existing frameworks. This iterative process, bridging theory and observation, is a cornerstone of scientific progress and fosters an increasingly sophisticated grasp of the universe.
3. Mathematical Rigor
Mathematical rigor is inextricably linked to scientific advancements, particularly within theoretical physics. The precision and sophistication of mathematical tools are essential for formulating and testing theories, including those related to the subject of interest. Rigorous mathematical frameworks provide a structured approach to describing the universe's complexities, enabling predictions and facilitating the evaluation of theoretical models. This connection is particularly evident in the work of renowned scientists, highlighting the importance of mathematical tools for advancing scientific understanding.
- Formalization of Concepts
Mathematical rigor facilitates the formalization of concepts. Abstract ideas, like spacetime curvature in general relativity or quantum entanglement, are translated into precise mathematical structures. This formalization allows for a deeper understanding of underlying principles. Formulas and equations offer a standardized way to express complex ideas, providing unambiguous definitions and enabling precise calculations. This is crucial for evaluating the consistency and potential ramifications of such concepts.
- Predictive Power
Mathematical rigor is fundamental to the predictive power of theories. Precise mathematical formulations enable the derivation of equations and relationships that describe the behavior of systems. This allows scientists to make predictions about future events or phenomena, which can then be tested through observation. The accuracy of these predictions is directly linked to the rigor of the underlying mathematical framework. For instance, the precise predictions of general relativity, based on rigorous mathematical models, have been confirmed through observations of gravitational lensing and other phenomena.
- Testing Theoretical Frameworks
Mathematical models provide a framework for rigorously evaluating theoretical frameworks. The consistency and internal coherence of a theory can be meticulously assessed through mathematical analysis. Mathematical tools can identify inconsistencies or limitations within a theoretical model. By using rigorous mathematical frameworks, scientists can determine whether a theoretical model is self-consistent and whether the model accurately accounts for observed data, or if it requires further refinement or revision.
- Quantifying and Measuring Phenomena
Mathematical rigor allows for precise quantification and measurement of physical phenomena. Mathematical equations often define relationships between measurable quantities, providing a basis for comparing theoretical predictions with experimental results. This quantification is essential for analyzing and interpreting data from various scientific experiments and observations, supporting the verification of theoretical models. This accuracy in measurement is vital to establishing the robustness and reliability of the mathematical framework.
In summary, mathematical rigor is a cornerstone of scientific advancement, particularly in theoretical physics. The precise formulation of concepts, the predictive power of models, the evaluation of theories, and the accurate measurement of phenomena all depend on the application of mathematical rigor. Through this combination of abstract thinking and precise calculations, scientists can develop models and formulate theories that further our understanding of the universe and its governing principles.
4. Scientific Methodology
Scientific methodology, encompassing systematic observation, experimentation, and analysis, underpins the pursuit of knowledge, including the work of significant figures in science. This methodical approach ensures rigor and reliability in the process of generating knowledge, allowing for a degree of objectivity and preventing subjective bias from influencing the interpretation of findings. Critical to this process is the formulation of testable hypotheses, the design of controlled experiments, and the careful analysis of data, enabling scientists to draw valid conclusions from observations. Without adherence to these principles, scientific advancement would be significantly hampered, potentially leading to erroneous interpretations and a lack of progress in understanding the natural world.
The application of scientific methodology is evident in numerous scientific endeavors. For example, the development of the theory of evolution by natural selection relied heavily on meticulous observation of diverse species and analysis of fossil records. The rigorous methodology employed in this process facilitated the formulation of a theory that continues to influence biological and environmental studies. Similarly, the investigation into the properties of light, culminating in the development of quantum mechanics, benefited greatly from carefully controlled experiments and rigorous mathematical analyses. This meticulous approach highlights the importance of adhering to established methodology to advance scientific knowledge. Scientific methodology provides a framework for minimizing errors in experimentation and data analysis, allowing for robust and reliable results.
In conclusion, scientific methodology is not merely a set of procedures but a fundamental principle underlying scientific progress. Adherence to this methodology is crucial for generating reliable and robust knowledge, especially in the pursuit of complex phenomena. The successful application of scientific methodology facilitates a deeper understanding of the natural world, driving innovation and progress across various disciplines. The exploration of any scientific concept, regardless of its complexity, should rely on the foundation of rigorously applied scientific methodology.
5. Predictive Accuracy
Predictive accuracy is a critical component in scientific endeavors, particularly in fields like theoretical physics. Accuracy in prediction directly correlates with the robustness and validity of a scientific model. The ability to accurately forecast outcomes, based on established theories, allows for verification, refinement, and expansion of existing knowledge. In the context of groundbreaking work, like that of notable scientists, predictive accuracy becomes a cornerstone for validating complex models and theories, crucial to scientific progress.
- Validation of Theoretical Frameworks
Accurate predictions arising from a theory lend strong support to the validity of the underlying framework. If predictions consistently match observed phenomena, the model gains credibility. The ability to successfully anticipate outcomes in diverse scenarios bolsters confidence in the theoretical model. This process is crucial for accepting a theory as a reliable tool for understanding and analyzing physical systems. Examples include the successful predictions of gravitational lensing based on Einstein's theory of general relativity, which significantly strengthened the theory's acceptance.
- Refinement and Adaptation of Models
Discrepancies between predictions and observations serve as crucial indicators for model refinement. When predictions fail to align with empirical data, scientists identify areas requiring adjustments and modifications. This iterative process is essential for the development of more precise and accurate models, leading to progressive advancements in understanding the natural world. The theory of black holes, for example, has evolved as observational data has improved, leading to refinements of existing models.
- Motivation for Further Research
Successful predictions stimulate further research and exploration. Accurate anticipations of future outcomes encourage scientists to delve deeper into the underlying mechanisms or to explore related phenomena. Precise predictions create avenues for testing the boundaries of a theory, leading to a more thorough understanding and a widening scope of applications. The possibility of early detection of phenomena using precise predictions, such as gravitational waves, encourages greater research in related areas of physics.
- Driving Technological Advancement
Accurate predictions often pave the way for technological advancements. Understanding physical phenomena accurately allows engineers to create technologies that harness these principles. Predictive models facilitate advancements in diverse fields such as communication, medicine, and materials science, with consequences for broader societal improvement. Accurate predictions regarding the behavior of materials, like those in high-energy physics, are foundational to the advancement of technologies and materials, leading to tangible improvements in daily life.
In conclusion, predictive accuracy is a fundamental aspect of scientific progress. The ability to anticipate outcomes strengthens the validity of models and fuels further research. The accuracy of predictions plays a pivotal role in refining our understanding of physical phenomena and driving innovation across various disciplines. This is demonstrably evident in the continuous evolution of scientific thought, and especially relevant to the intellectual inquiries of key figures in science.
6. Societal Influence
Societal influence significantly impacts scientific inquiry. This influence manifests in various ways, including funding priorities, public perception, and ethical considerations. The pursuit of knowledge, epitomized by figures like Stephen Hawking, is rarely isolated from the societal context in which it occurs. Funding agencies, for example, often allocate resources based on perceived societal needs or priorities. A robust economy, with a supportive research infrastructure and funding for scientific advancement, facilitates the investigation and development of complex theories. Conversely, societal indifference or disinterest can limit resources and hinder progress. Public perception of scientific endeavors, often shaped by media representation and public discourse, can influence research directions and public support for scientific initiatives.
The accessibility of scientific knowledge and communication play a crucial role. Effective dissemination of research findings, whether through publications, public lectures, or educational outreach, shapes public understanding and can inspire further interest in science. The societal response to scientific discoveries can influence subsequent research. For instance, public concerns about climate change have driven greater investment and research into climate modeling and renewable energy. Conversely, the lack of societal awareness or concern can lead to limited funding for relevant research areas. Furthermore, the ethical considerations surrounding certain scientific advancements, like genetic engineering or artificial intelligence, are often heavily influenced by public discourse, shaping the trajectory of research and potentially limiting exploration. The example of Stephen Hawking's work on black holes and cosmology, while theoretically driven, has always had a profound societal impact through accessible explanations, influencing public awareness and fascination with the universe.
Understanding the interplay between societal influence and scientific inquiry is crucial. It enables a more nuanced appreciation of the factors that shape scientific progress. By considering the impact of funding, public perception, ethical considerations, and knowledge dissemination, scientists, policymakers, and the public can collaboratively cultivate a supportive environment for scientific advancement, fostering a more comprehensive approach to the pursuit of knowledge. This understanding is paramount in making informed decisions about research priorities and ensuring that scientific endeavors effectively address societal challenges and needs, while maintaining ethical and responsible practices.
7. Accessibility to Knowledge
Accessibility to knowledge is a critical component of scientific progress, particularly when considering figures like Hawking. The dissemination of findings, theories, and research methodologies is essential for the advancement of knowledge. Without the ability for researchers to access and share information, discoveries might remain isolated, hindering the development of broader understanding. Hawking's work, for example, significantly benefited from and contributed to this accessibility. His books, lectures, and public engagement brought complex scientific concepts to a wider audience, fostering interest in theoretical physics and cosmology. This accessibility spurred further study and research, creating a ripple effect of intellectual growth and innovation.
The practical significance of this understanding is profound. Open access to knowledge facilitates collaboration across geographical and institutional boundaries. Researchers can build upon existing findings, identify gaps in knowledge, and develop innovative solutions through shared understanding and collaborative efforts. This interconnectedness accelerates scientific progress and leads to more comprehensive models and theories. The ability to easily access and share research findings empowers a global community of scientists, promoting innovation and problem-solving on a grander scale. Moreover, public access to knowledge can spark interest, encouraging new generations to pursue careers in science, technology, engineering, and mathematics (STEM). Hawking's efforts in popularizing scientific concepts exemplify this critical link between accessibility and societal engagement.
In conclusion, accessibility to knowledge is not merely a desirable characteristic but a crucial driver for scientific advancement. The ability to share, understand, and build upon existing information is essential for the continued progress of fields like theoretical physics. This principle, exemplified by figures like Hawking, highlights the interconnectedness of knowledge and emphasizes the need for open access, dissemination, and effective communication to further the pursuit of scientific understanding across disciplines and cultures. Obstacles to accessibility, such as language barriers or financial limitations, hinder this progress and necessitate concerted efforts to ensure equitable access for all.
Frequently Asked Questions about Hawking
This section addresses common inquiries about the life and work of Stephen Hawking. The answers are grounded in factual information and aim to clarify key aspects of his contributions to science and public discourse.
Question 1: What were Stephen Hawking's most significant contributions to science?
Hawking's contributions primarily lie in theoretical physics, particularly cosmology and black hole research. His most notable achievements include developing theoretical models of black hole thermodynamics and the singularity theorems, which provided compelling evidence for the universe's origin in a singularity. His work also explored the relationship between general relativity and quantum mechanics, bridging seemingly disparate fields of study.
Question 2: How did Hawking's physical condition impact his work?
Hawking's debilitating motor neuron disease, ALS, significantly impacted his physical capabilities. However, it did not impede his intellectual pursuits. He utilized advanced communication aids and dedicated collaborators to convey his groundbreaking research to the scientific community and the public. His determination and resilience in the face of adversity serve as an inspiration.
Question 3: What was the significance of Hawking's book "A Brief History of Time"?
"A Brief History of Time" achieved unprecedented success in popularizing complex scientific concepts. It conveyed the mysteries of the universe, including black holes and the Big Bang, to a wider audience. This accessibility fostered public interest in scientific pursuits and provided a glimpse into the complexities of theoretical physics.
Question 4: What was Hawking's stance on the possibility of extraterrestrial life?
Hawking expressed a degree of cautious optimism about extraterrestrial life. He believed that the sheer size of the universe and the multitude of stars made the existence of other forms of life highly probable, but he also acknowledged the inherent challenges in detecting such life and the potential risks involved.
Question 5: How did Hawking's work influence public understanding of science?
Hawking's work profoundly influenced public understanding of science. His accessible writing and public engagement effectively broke down complex scientific theories for a broader audience. His influence inspired many, particularly young people, to pursue careers in science and technology.
In summary, Stephen Hawking's legacy extends beyond his scientific achievements. His resilience, his communication, and his ability to connect complex concepts to a wider audience made him a truly influential figure in science and society.
The subsequent section will explore the broader impact of Hawking's work on contemporary scientific discourse.
Conclusion
This exploration of Hawking's work reveals a multifaceted legacy. The article highlights the profound impact of theoretical models, emphasizing their role in generating predictions and fostering a deeper understanding of complex phenomena. Observational evidence, crucial for validating theoretical frameworks, was examined, showcasing the iterative interplay between abstract reasoning and empirical data. The essential role of mathematical rigor in the formulation and testing of theories was also underscored, demonstrating its importance in scientific advancements. Further, the article emphasized the influence of scientific methodology, its ability to promote objectivity and prevent bias, and the profound significance of predictive accuracy in verifying theoretical models and driving further research.
Hawking's contributions are not confined to the realm of theoretical physics. The societal impact of accessible scientific knowledge, facilitated by clear communication, was explored. This accessibility fosters public engagement and inspires future generations to pursue scientific careers. The discussion also underscores the significance of accessibility to knowledge, facilitating collaboration and accelerating scientific progress. The frequently asked questions section further clarifies key aspects of Hawking's life and work, showcasing his dedication to scientific inquiry and his enduring influence on public understanding of complex scientific concepts. In conclusion, Hawking's legacy stands as a testament to the enduring power of rigorous scientific methodology, the transformative potential of accessible knowledge, and the crucial role of unwavering intellectual curiosity in shaping human understanding of the universe.
- Discovering The Wonders Of Victoria Falls Best Times To Visit
- Understanding The Dual Price System A Comprehensive Guide
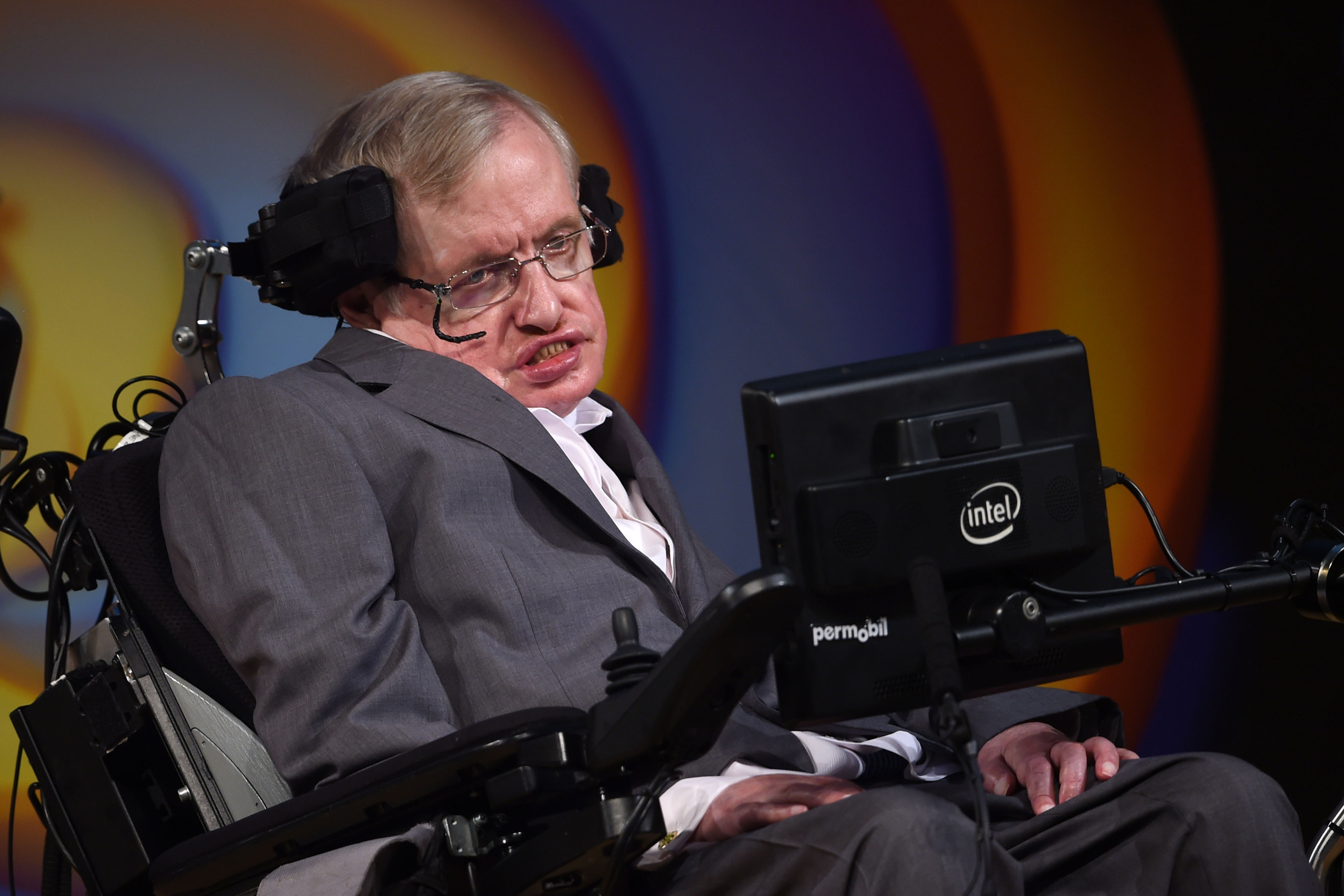
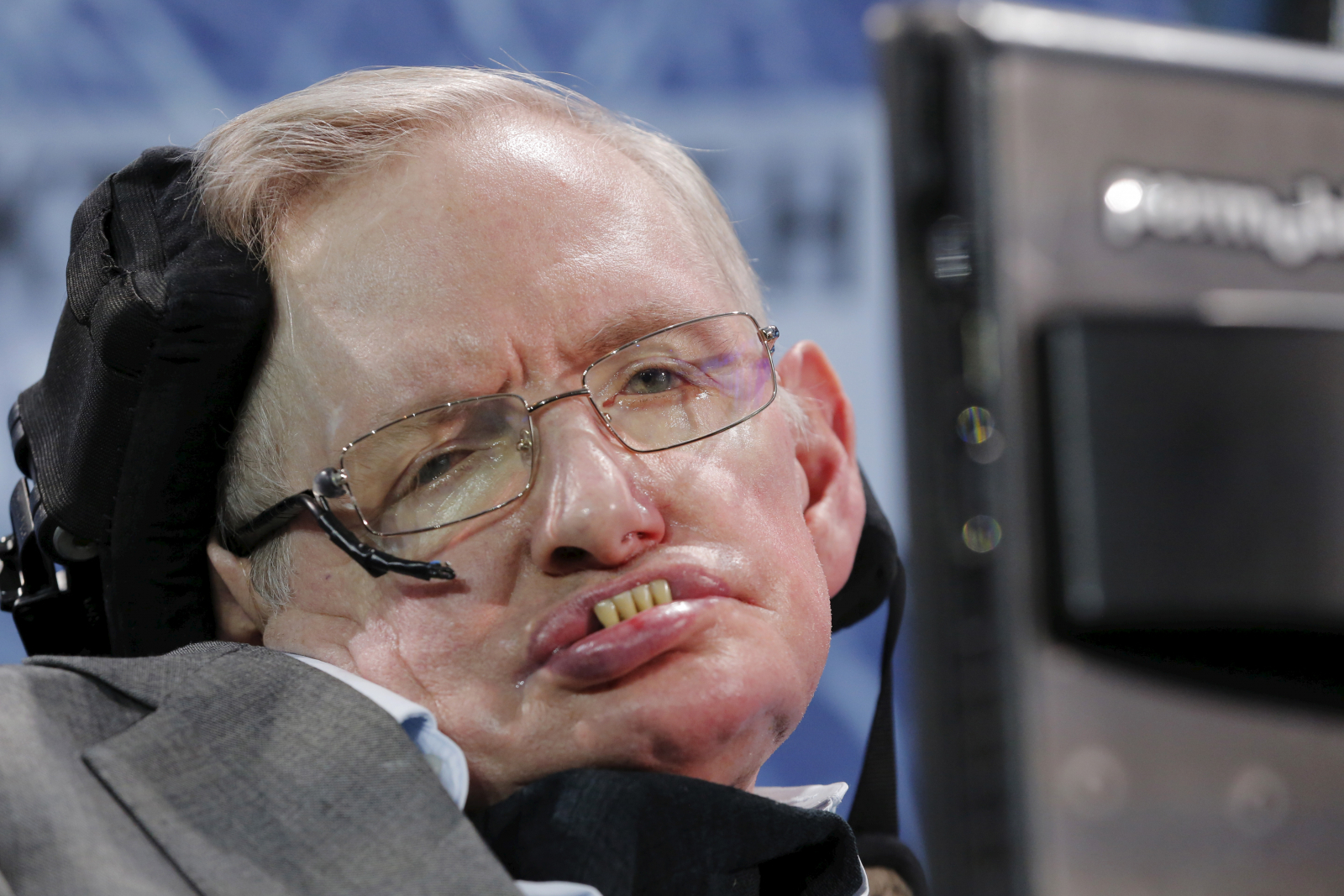
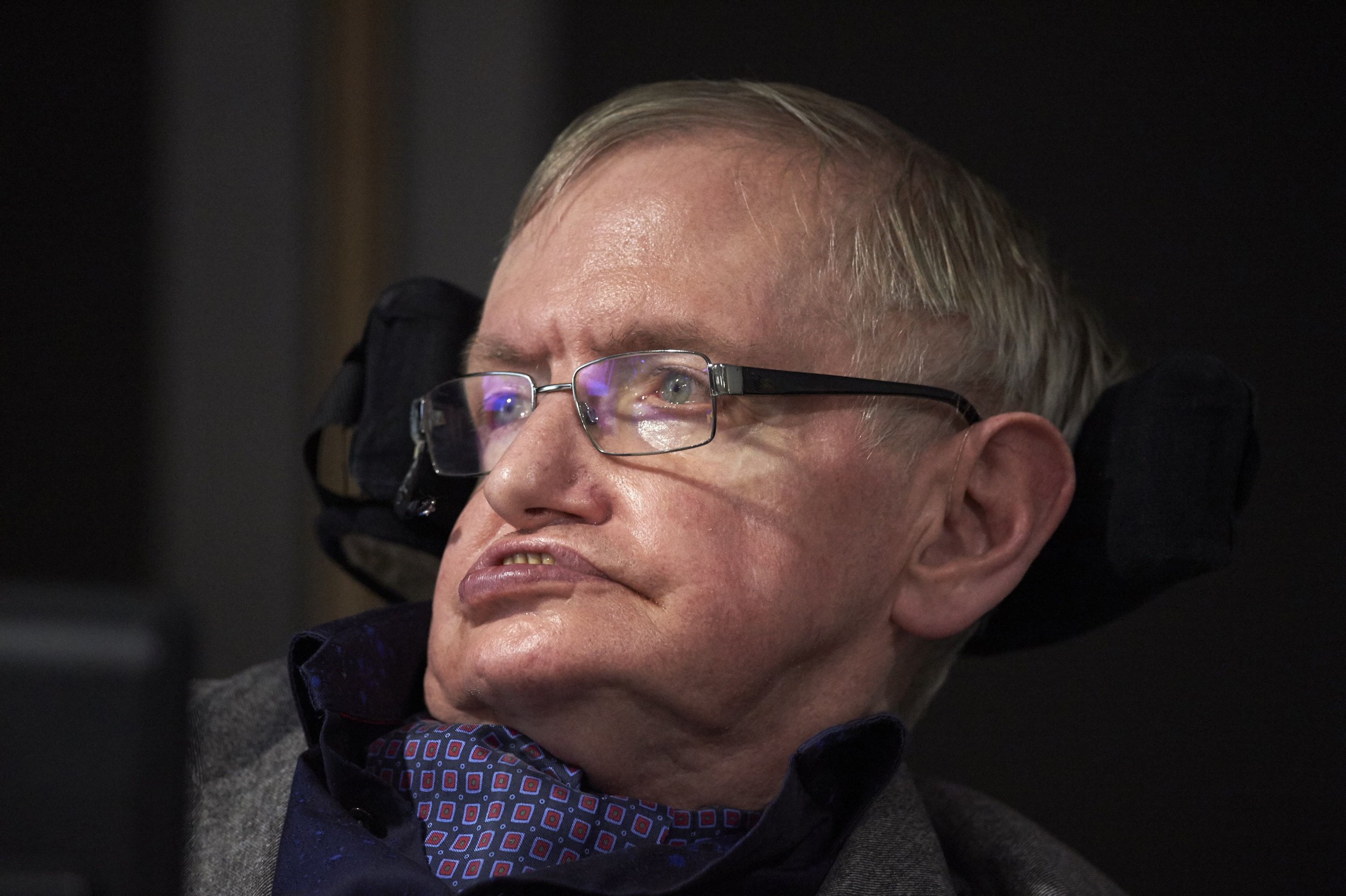